Experimental Nuclear and Particle Physics
The Department of Physics has an active and widely recognized program in Nuclear and Particle Physics. The majority of our present experimental programs is focused at Jefferson Laboratory in Newport News, Virginia, which is an international center for nuclear physics research. In addition, we are engaged in a program of fundamental physics using cold neutrons at Los Alamos and Oak Ridge national Laboratories.
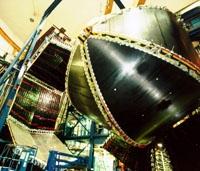
The Department of Physics has an active and widely recognized program in Nuclear and Particle Physics. The majority of our present experimental programs is focused at Jefferson Laboratory in Newport News, Virginia, which is an international center for nuclear physics research. In addition, we are engaged in a program of fundamental physics using cold neutrons at Los Alamos and Oak Ridge national Laboratories.
At Jefferson Lab, we are embarking on an ambitious experimental program that will search for the "heavy photon", a partner to the photon and a dark matter candidate. The heavy photon search (HPS) experiment will use an uncommonly small, almost table top, detector at the intensity frontier in particle physics.
We also lead a program at Jefferson Lab for studying the structure of the nucleon through spin-dependent observables. As part of this effort, we are analyzing the data from the recently completed g2p experiment, which should shed light on the so called `Proton Radius Puzzle'. Our group is is also at the forefront of an exciting new effort to measure Tensor Spin Observables using a novel tensor polarized target.
At Los Alamos and Oak Ridge we are collaborating on experiments using newly available beams of very slow neutrons to study the properties of the neutron itself. These experiments are sensitive to the fundamental interaction between the constituent quarks within the neutron and to the fundamental interaction leading to its beta decay to become a proton.
We had a major role in the design, construction, and commissioning of a major instrument for nuclear and nucleon physics called BLAST. It used the 1 GeV electron beam at the Bates accelerator as a means to examine matter and fields on a scale ten thousand times smaller than the atom. The BLAST detector has now been disassembled and shipped to Germany, where it was used in the Olympus experiment at DESY.
Professors: John Calarco, Maurik Holtrop, Karl Slifer, Patricia Solvignon
Nuclear and High Energy Theory
The research of the nuclear and high energy theory group ranges from the study of nuclear structure and Quantum Chromodynamics (QCD) to string theory. Prof. Heisenberg's nuclear structure simulation project explores how shapes, sizes, and interior density distributions of normal nuclei arise from the known forces between nucleons. Computer codes keep track of how strongly each combination of two particles interact with each other in all possible configurations. They also include the effects of how a third particle nearby can modify the force between those two particles. The stable configurations of nuclei assembled from sixteen particles can be calculated from all these interactions, two (or three) at a time. His program excels at determining and comparing the spatial relationships between the nucleons in the various low-lying nuclear energy levels.
The research of the nuclear and high energy theory group ranges from the study of nuclear structure and Quantum Chromodynamics (QCD) to string theory. Prof. Heisenberg's nuclear structure simulation project explores how shapes, sizes, and interior density distributions of normal nuclei arise from the known forces between nucleons. Computer codes keep track of how strongly each combination of two particles interact with each other in all possible configurations. They also include the effects of how a third particle nearby can modify the force between those two particles. The stable configurations of nuclei assembled from sixteen particles can be calculated from all these interactions, two (or three) at a time. His program excels at determining and comparing the spatial relationships between the nucleons in the various low-lying nuclear energy levels.
Professor Beane is currently involved in theoretical research whose ultimate goal is to establish contact between nuclear and hadronic physics and Quantum Chromodynamics (QCD) --- the gauge field theory of quarks and gluons that underlies all of hadronic and nuclear phenomena. QCD is very hard to solve analytically; in fact very little is known about the exact solution. However, there has recently been remarkable progress in simulating QCD with computers using lattice gauge theory, which involves replacing space-time with a grid and using Montecarlo numerical-integration methods. The lattice QCD simulations are carried through using unphysically-large values of the quark masses. Furthermore, currently-utilized lattice spacings /lattice sizes are not much smaller/larger than characteristic physical length scales of interest, like the size of the proton. Fortunately one can formulate continuum effective quantum field theories which allow one to extrapolate from the unphysical quark masses, lattice spacings and lattice volumes to nature in a rigorous manner. The controlled theoretical error analysis provided by effective field theories is particularly crucial for searches for physics beyond the Standard Model of particle physics involving hadronic and nuclear experiments, and for the hadronic and nuclear input often required in astrophysics and cosmology. Professor Beane has been especially interested in making contact between the simplest nuclear systems, which involve two nucleons, and QCD using lattice methods. It turns out that the dependence of the two-nucleon systems (for instance the deuteron binding energy) on the quark masses that appear in the QCD lagrangian involves a low-energy parameter that cannot be determined from experiment. However, a lattice QCD simulation over a range of quark masses would enable a determination of the low-energy two-nucleon S-matrix as well as the parameter governing the quark-mass dependence. Knowledge of the quark mass dependence is not strictly academic as it would allow one to place bounds on the time-dependence of fundamental parameters --- like the Higg's vacuum expectation value--- using big-bang nucleosynthesis. These ideas have been bolstered by recent observations of distant quasars which suggest that the fine-structure constant was smaller in the distant past than it is today.
Professor Dawson calculates the properties of matter under the influence of QCD at very high temperature. These conditions probably existed a short time after the birth of the universe, and will soon be simulated with a new accelerator. These calculations will help us determine whether strongly interacting nuclear particles can undergo a phase transition at high temperature, radically changing their properties, much the way water boils and becomes steam. In the normal phase the quarks and gluons that comprise the internal structure of protons and neutrons are confined to stay inside. This new phase, called the quark-gluon plasma, would be a liberation of these internal constituents. The calculations will help us plan our measurements to determine whether the quark-gluon plasma occurs, and characterize its properties.
Professor Berglund studies string theory, the leading candidate for a theory of all the forces in nature. String theory employs one-dimensional objects, strings, as the fundamental building blocks and gives a unified description of the standard model of particle physics (quantum electrodynamics (QED), the weak interaction and QCD) and quantum gravity. In doing so, the theory predicts that the universe has more than four spacetime dimensions. These extra dimensions, however, play an important role in issues such as
- the origin of matter
- the different energy scales of the standard model of particle physics and quantum gravity
- the small size and positive nature of the cosmological constant.
Research in string theory at UNH focuses on addressing these problems by studying the properties of the extra dimensions.
Professors: Jochen Heisenberg (emeritus) , Silas Beane, John Dawson (emeritus), Per Berglund